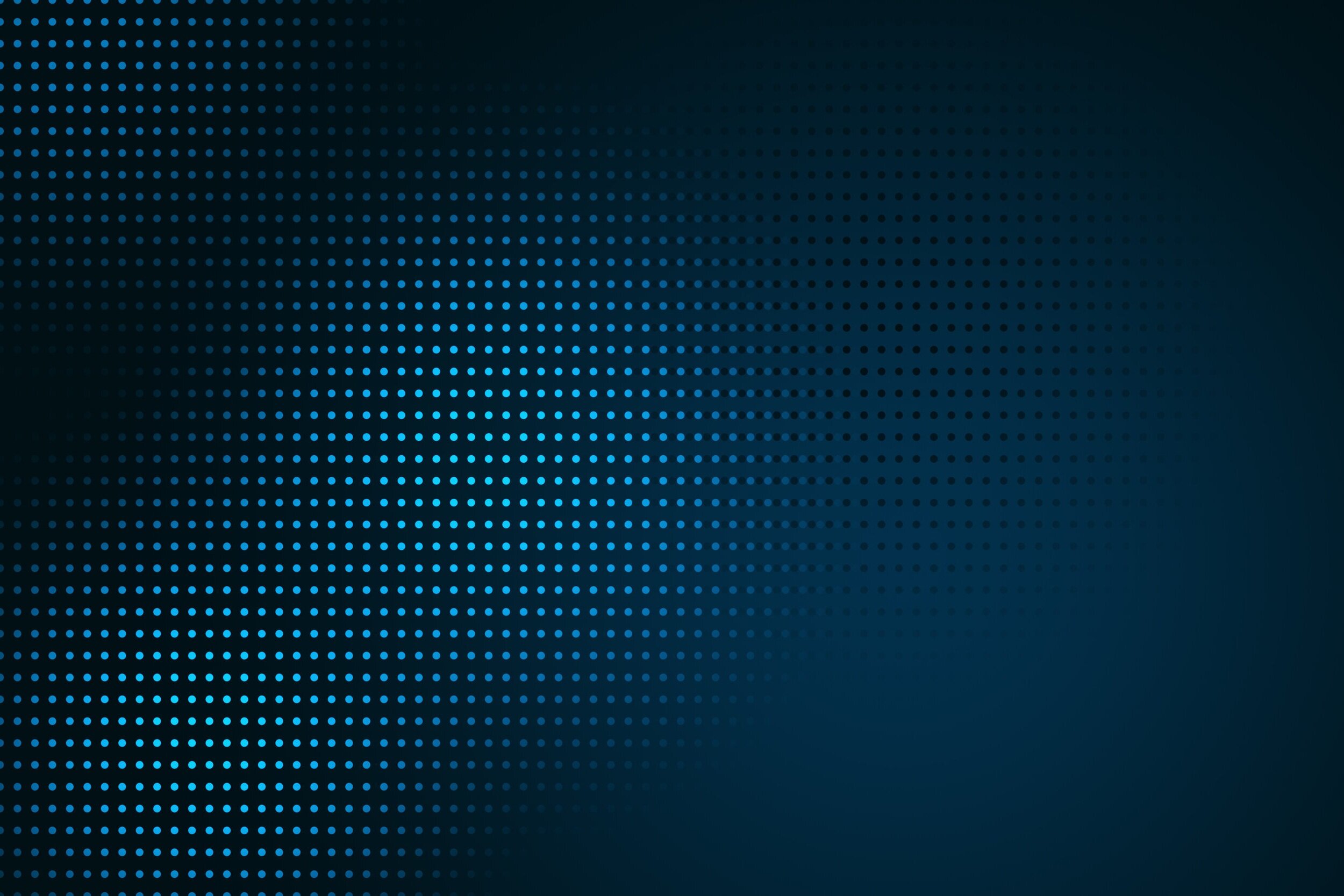
Research
Overview
Reducing carbon emission and ending the fossil fuel economy require the implementation of clean energies. Energy storage devices will be the most important infrastructures to power up future smart and resilient cities, where high energy, high power, high mobility, and reliable safety are highly demanded. Our research interests cover different energy storage chemistry for various application scenarios, including, but not limited to, metal batteries (Li, Na, etc), all solid state batteries, multivalent-ion batteries and so on.
Our research is at the core of materials science by bridging concepts from analytical chemistry and electrochemistry, and hold technological prospects in multiple engineering disciplines, including two primary directions:
(1) Developing multi-scale quantitative characterization tools, to answer key scientific questions in energy and environment related fields;
(2) Developing new materials, precision fabrication/manufacturing methods, and system integration, guided by the fundamental understanding from quantitative characterization to manipulate molecular interactions at dynamic interfaces and innovate sustainable technologies.
Anode-Free Lithium Metal Batteries
In commercial lithium ion batteries, lithium containing cathode provides the capacity, while graphite anode only serves as a host of lithium ions for safety considerations. Getting rid of the graphite anode, also called anode-free lithium metal battery (AFLMB), opens the opportunity to achieve a cell level energy density of 500 wh/kg and higher. The essence of AFLMB is to enable dendrite-free and high-efficient electrochemical cycling of lithium metal, requiring synergistic efforts of novel electrolyte design (both solid and liquid), fundamental understanding of lithium electrochemical behavior and system integration.
Reference: “Key issues hindering a practical lithium-metal battery”, Trends in Chemistry, 2019, 1, 152-158
Characterization by Design
Titration Gas Chromatography
Inactive or “dead” Li formation is the immediate cause of capacity loss and safety hazards of high-energy lithium metal batteries; it consists of both (electro)chemically formed Li+ compounds in the solid electrolyte interphase (SEI) and electrically isolated unreacted metallic Li. However, quantitatively distinguishing between Li+ in SEI components and the unreacted metallic Li has not been possible due to the lack of effective diagnosis tools. We established a new analytical method, TGC, and accurately quantified the contribution from unreacted metallic Li to the total amount of inactive Li. We identify the unreacted metallic Li, rather than the (electro)chemically formed Li+ in SEI, as the dominant source of inactive Li and capacity loss.
Reference: “Quantifying inactive lithium in lithium metal batteries”, Nature, 2019, 572, 511–515
Cryogenic Transmission Electron Microscopy
Cryo-TEM is a powerful tool to probe the nanostructures of electrochemcially active materials, such as lithium metal and its SEI. It is impossible to acquire high-resolution images of these materials at room temperature due to their extremely sensitive nature to electron beam. The cryogenic protection minimizes the beam damage to the brittle materials while preserve its intrinsic properties.
Reference: “Quantifying inactive lithium in lithium metal batteries”, Nature, 2019, 572, 511–515
Cryo-EM facility at MSU: Center for Advanced Microscopy
Cryogenic Focused Ion Beam
FIB-SEM images provide the cross-section morphology information of materials. Cryogenic protection is critical because the electrochemically active materials are not only sensitive to electron beam, but also is apt to react with the FIB incident ion beam at room temperature. Taking a series of cross-sectional FIB-SEM images, a 3D structure can be reconstructed, enabling 3D visualization and quantitative structural analysis.
Reference: “Pressure-tailored lithium deposition and dissolution in lithium metal batteries”, 2020, under review
Cryo-FIB facility at MSU: Composite Materials and Structures Center
Materials by Design
Surface Modification
Graphene oxide/polydopamine-coated Si nanocomposite (GO/PDA-Si) was synthesized by a novel facile solution-based chemical method at room temperature. The surface property of Si nano particles (NPs) was modified by introducing secondary amine groups from PDA, which form amide groups with carboxyl groups and hydrogen bonds with hydroxyl/carboxyl groups on GO. These chemical interactions firmly anchor Si NPs to GO so that aggregation of Si NPs can be mostly prevented.
Reference: “Improving the electrochemical performance of Si nanoparticle anode material by synergistic strategies of polydopamine and graphene oxide coating”, Journal of Physical Chemistry C, 2015, 119 (4), 1720–1728
Bulk Doping
Oxygen-redox reactions in lithium-rich layered oxide cathode materials enable ultra high capacity, but causes voltage decay due to the unwanted oxygen gas formation. We firstly synthesized Li[Li0.2Ni0.2Mn0.6]O2 cathode materials by the modified co-precipitation method. Guided by the ab initio calculations of oxygen vacancy formation energy, we then selectively chose the Co and Mo co-doping into the Li[Li0.2Ni0.2Mn0.6]O2 materials with the aims to facilitate oxygen activity while mitigate the voltage decay. The co-doping design enhances both capacity and cycling stability.
Reference: “Modified co-precipitation synthesis of meso-structure controlled Li-rich layered oxides for minimizing voltage degradation”, ACS Applied Energy Materials, 2018, 1, 3369
“Mitigating oxygen release in anionic-redox-active cathode materials by cationic substitution through rational design”, Journal of Materials Chemistry A, 2018, 6, 24651